Our research is aimed at obtaining molecular and mechanistic insights into fundamental cellular processes underlying human health and disease. To achieve this, we combine modern structural biology techniques, in particular single-particle cryo-electron microscropy and cryo-electron tomography, with biochemical, biophysical, cell biological and in vivo methods. This enables us to visualize molecular machineries that carry out essential tasks in eukaryotic cells and organelles at near-atomic resolution and study their structure and function from the atomic to the cellular scale (Figure 1).
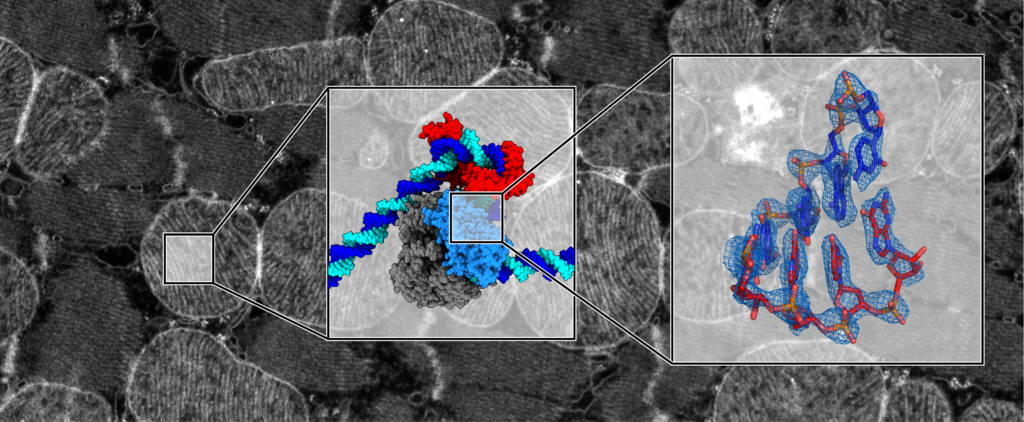
Our main research focus lies on mitochondria and their gene expression system. These double-membrane enclosed organelles within eukaryotic cells are often referred to as the ‘powerhouses’ of the cell, because the respiratory chain at their inner membrane provides the majority of chemical energy required for life. In addition, they play important roles in other processes such as metabolism, signaling and immune defense. Impaired mitochondrial function is associated with ageing, and mitochondrial disorders are among the most common inheritable diseases.
As a remainder of their endosymbiotic origin, mitochondria maintain their own genome (mtDNA) which encodes for essential components of the respiratory chain. In order to form functional complexes, these subunits must assemble with a large number of nuclear-encoded subunits that are imported into the organelle. Therefore, the expression of mitochondrial genes needs to be regulated as well as coordinated with nuclear gene expression to ensure proper mitochondrial function. The importance of this process is underscored by the fact that defects in mitochondrial gene expression lead to severe disease in humans. Mitochondrial gene expression is carried out by a set of dedicated molecular machineries, which include a mitochondrial RNA polymerase and a mitochondrial ribosome. Over the course of evolution, this apparatus has adopted features found in nuclear, bacterial, and viral gene expression systems, thus making it truly unique. Despite its fundamental role in cellular function, the molecular mechanisms underlying mitochondrial gene expression and how it is regulated in response to varying cellular needs are not well understood.

The research in our group addresses fundamental open questions in mitochondrial molecular biology. Our goal is to obtain a molecular understanding of how the human mitochondrial genome is expressed, how this process is regulated and coordinated, and how it is embedded in a cellular context. To achieve this, we study both the molecular mechanisms underlying mitochondrial gene expression as well as how individual processes are coordinated, coupled and organized within the organelle. In particular, we combine in vitro (single-particle cryo-EM, X-Ray crystallography) with in situ (cryo-electron tomography) structural biology to visualize the macromolecular complexes that carry out mitochondrial gene expression (Figure 2) and dissect their mechanism of action. In the long term, this will provide important molecular insights into mitochondrial function in human health and disease.